Last June, I participated in a four-hour session on fusion energy during SF DeepTech Week at the SVB Experience Center in San Francisco. It was an electrifying event, showcasing the latest breakthroughs in fusion technology, the ambitious work of innovative startups, and even the groundbreaking findings from Lawrence Livermore National Laboratory, a major milestone in achieving energy balance. In this blog, I’ll dive into why investors are increasingly seeing fusion energy as a pivotal opportunity driven by innovation, macroeconomic shifts, and political alignment.
Today, fusion energy is moving beyond its traditionally niche status, with new macro-drivers giving it fresh momentum. Yet, with all the hype, a fundamental question remains: Is fusion still the elusive "20 years away" technology, or is it finally here to stay? Investors face a paradox. On one hand, fusion is often perceived as high-risk, slow to deploy due to intense regulatory hurdles, and potentially hazardous at first glance. On the other, it holds unparalleled potential to transform the clean energy landscape, offering an alternative to fossil fuels without the intermittency challenges of renewables.
At its core, fusion energy aims to replicate the sun’s power here on Earth. But how do we build a miniature star? The key is achieving incredibly high temperatures—around 14 million degrees Celsius—hot enough to strip electrons from atoms, creating a plasma where nuclei and electrons move freely. Since atomic nuclei naturally repel each other, they need to collide at extreme speeds to overcome this barrier and fuse, made possible by high temperatures. In stars, immense gravitational pressure helps force these nuclei together, triggering fusion and releasing vast amounts of energy. The challenge for scientists and engineers is replicating this process under controlled conditions to create a viable energy source.
Currently, there are two main types of fusion reactors:
- Magnetic Confinement Reactors: These use powerful electromagnetic fields to contain plasma in a doughnut-shaped chamber. The most well-known designs include tokamaks and stellarators.
- Inertial Confinement Reactors: These rely on high-energy pulses, typically from powerful lasers, to heat the surface of a fuel pellet, causing it to implode and initiate fusion. Some variations use lasers, X-pinch or Z-pinch methods to achieve similar results.
- Magnito-Inertial Confinement: A mixture of 1 and 2.
Fusion Energy in a Historic Context
Fusion energy’s story began in the early 20th century when Sir Arthur Eddington proposed that stars generate power through nuclear fusion. By the 1930s, Hans Bethe had detailed how the sun fuses atoms to release energy. But while nature had mastered fusion, replicating it on Earth remained elusive.
During World War II, the development of nuclear fission led to the hydrogen bomb, proving fusion’s immense power. The challenge then became controlling it for energy production. In the 1950s, early experiments like the UK’s ZETA and Soviet tokamaks attempted to confine superheated plasma with magnetic fields, while Lyman Spitzer’s stellarator offered an alternative approach. However, plasma proved difficult to contain.
By the 1980s, fusion had become a global effort, culminating in the formation of ITER, the world’s largest fusion experiment. Tokamaks like JET and TFTR made strides, while laser-based inertial confinement fusion advanced at Lawrence Livermore National Laboratory. Progress continued into the 2000s, with Germany reviving the stellarator concept and new superconducting magnets improving efficiency.
The 2010s saw a major shift as private investors, frustrated by slow progress, poured billions into fusion startups like Commonwealth Fusion Systems and Helion Energy. Today, with over $6 billion in private funding and rapid technological advancements, fusion’s long-standing reputation as "always 20 years away" may finally be changing.
The Fundamentals of Fusion Energy
Fusion energy replicates the process that powers stars, where hydrogen nuclei fuse to form helium, releasing enormous amounts of energy. This reaction is the most efficient energy generation process in nature. However, harnessing fusion on Earth is challenging. Fusion reactions reach a bottleneck at iron (Fe), the element where nuclear fusion ceases to produce net energy. Beyond iron, fusion requires more energy than it generates. The challenge lies in overcoming plasma instabilities and designing reactors that make fusion viable, scalable, and sustainable.
Types of Fusion Technologies
Magnetic Confinement Fusion (MCF)
Magnetic Confinement Fusion (MCF) uses powerful magnetic fields to confine and control plasma at the extreme temperatures and pressures required for fusion. This approach prevents the plasma from touching the reactor walls while maintaining conditions necessary for sustained reactions.
Tokamak
The tokamak is the most widely studied fusion reactor design. It uses a doughnut-shaped (toroidal) chamber and a combination of electric currents and magnetic fields to confine plasma. Tokamaks have demonstrated significant progress in sustaining plasma and generating fusion power, but they require advanced superconducting magnets and precise control to maintain stability.
Examples: ITER, Commonwealth Fusion Systems
Stellarator
A variation of the tokamak, the stellarator, uses twisted magnetic fields to improve plasma stability and reduce energy losses. This design eliminates the need for strong plasma currents, making it inherently more stable but also more complex to build. Advances in computational modeling have helped revive interest in stellarators as a potential alternative to tokamaks.
Examples: Wendelstein 7-X, Proxima Fusion
Emerging Players in MCF
Several companies are pushing forward with innovations in MCF, including ENN Group, OpenStar Technologies, Gauss Fusion, Stellarex, Realta Fusion, Energy Singularity, nT-Tao Compact Fusion Power, Type One Energy, Thea Energy, Deutelio, Novatron Fusion Group, and Helical Fusion.
Inertial Confinement Fusion (ICF)
Inertial Confinement Fusion (ICF) relies on using intense bursts of energy to rapidly compress fusion fuel, achieving the extreme densities and temperatures needed for fusion reactions. Unlike MCF, which maintains a continuous plasma state, ICF works by igniting small fuel pellets in rapid succession.
Laser-Driven Fusion
In laser-driven fusion, high-powered lasers focus energy onto a small pellet of deuterium and tritium, causing it to implode and trigger a fusion reaction. This approach has achieved significant breakthroughs but requires precision and massive energy inputs.
Examples: National Ignition Facility (NIF), Xcimer Energy
Electrostatic Confinement Fusion
Electrostatic confinement uses electric fields instead of magnetic fields to trap and accelerate plasma. This method is compact and suited for smaller-scale applications, but it has yet to demonstrate energy break-even on a commercial scale.
Examples: Avalanche Energy
Z-Pinch Fusion
Z-pinch fusion compresses plasma using an intense electric current, creating a self-generated magnetic field that confines the plasma. This method is relatively simple but historically unstable, though modern innovations are improving its viability.
Examples: Zap Energy
Alternative Fusion Approaches
Magnetized Target Fusion (MTF)
Magnetized Target Fusion (MTF) combines elements of both MCF and ICF by magnetically confining plasma and then compressing it with an external force. This hybrid approach aims to reduce complexity while maintaining efficiency.
Examples: General Fusion
Direct Energy Conversion
Instead of relying on traditional steam turbines, direct energy conversion methods extract energy from fast-moving charged particles produced during fusion reactions, significantly improving efficiency.
Examples: Helion Energy
High-Temperature Superconductors (HTS)
Superconducting magnets play a crucial role in magnetic confinement fusion, and the development of high-temperature superconductors (HTS) has made them more efficient and practical for long-term reactor designs.
Examples: Commonwealth Fusion Systems
Key Challenges for Fusion Technologies
Despite significant advancements, fusion energy still faces major hurdles:
- Net Energy Gain – The biggest challenge is achieving more energy output than input, a milestone that fusion researchers continue to pursue.
- Tritium Scarcity – Tritium, a crucial fusion fuel, is rare and requires sustainable breeding methods to support future fusion reactors.
- Engineering Complexity – Controlling plasma at extreme temperatures and pressures is a major technological challenge.
- Economic Viability – Fusion reactors must become cost-effective and scalable to compete with existing energy sources.
The Landscape of Fusion Startups
Fusion energy is no longer a distant dream—it’s becoming a reality thanks to startups leveraging innovative technologies and unique approaches. Below are the key players shaping the future of fusion energy:
1. Marathon Fusion
- Founder: Kyle Schyller
- Focus: Revolutionizing the efficiency of fusion power plants.
- Key Insights:
- A single fusion plant can produce 1 TWh annually, yet today’s energy demand exceeds 180,000 TWh.
- Key bottlenecks include tritium sustainability and scaling manufacturing to produce 100 units/day to reach 1M plants.
- Tritium, a critical fuel, must follow a self-sustaining path using three critical metrics: tritium breeding ratio, burn efficiency, and cycle time.
2. Commonwealth Fusion Systems (CFS)
- Founder: Darren Woufle
- Key Achievements:
- Raised $2B and employed 650 FTEs.
- Developing the SPARC reactor in collaboration with MIT.
- Utilizing high-temperature superconducting magnets, a breakthrough that allows more power out of plasma than energy input.
- High-performance tokamak reactors are the centerpiece, offering a leap over traditional designs.

3. Thea Energy
- Founder: David Gates
- Focus: Improving tokamak designs for better scalability and maintenance.
- Key Points:
- Raised $20M in a Series A round in 2023.
- Innovating modular designs to allow easier maintenance.
- Two-step energy production: DT fusion for initial power generation and scaling to broader energy outputs.

4. Avalanche Energy
- Founder: Robin Langtry
- Target Applications: Distributed energy, maritime power, and data centers.
- Roadmap:
- Progressing toward fusion densities of 10^12 plasma density/cm³ through incremental advancements.
- Focused on reducing barriers to testing, enabling rapid prototyping of ideas.
- Raised Series A in 2022.


5. Zap Energy
- Core Technology: Z-pinch magnetic confinement without superconducting magnets.
- Key Innovations:
- Cylindrical plasma confinement using magnetic instabilities (sausage shapes and kinks).
- A unique approach bypassing the need for solid walls to contain plasma, resulting in less material damage.
- 25 MW output per unit with 50 MW to the grid.
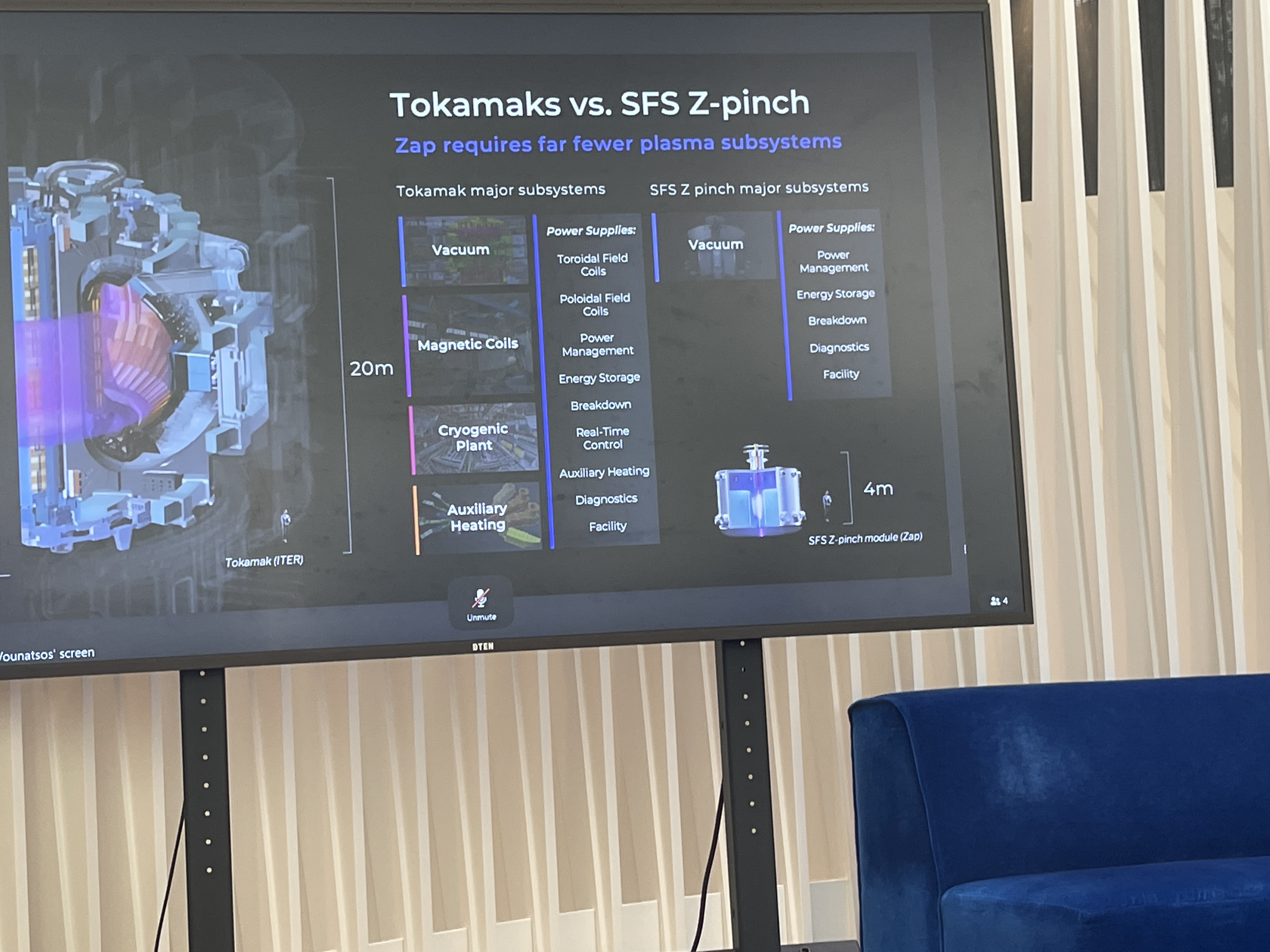
6. NIF and Lawrence Livermore’s Recent Breakthroughs
- Focus: Achieving ignition energy gains through laser-based inertial confinement.
- Milestones:
- 2.4x energy out vs. energy in, with goals to reach 75-100x for practical power plants.
- Innovations in higher-density fuels and symmetric confinement to improve energy output.

7. Xcimer Energy
- Focus: Laser-driven inertial confinement with advanced fuel compression systems.
- Key Features:
- Liquid walls and molten salt to protect reactor chambers and breed tritium.
- Raised $100M Series A for scaling up round-implosion-based laser systems.
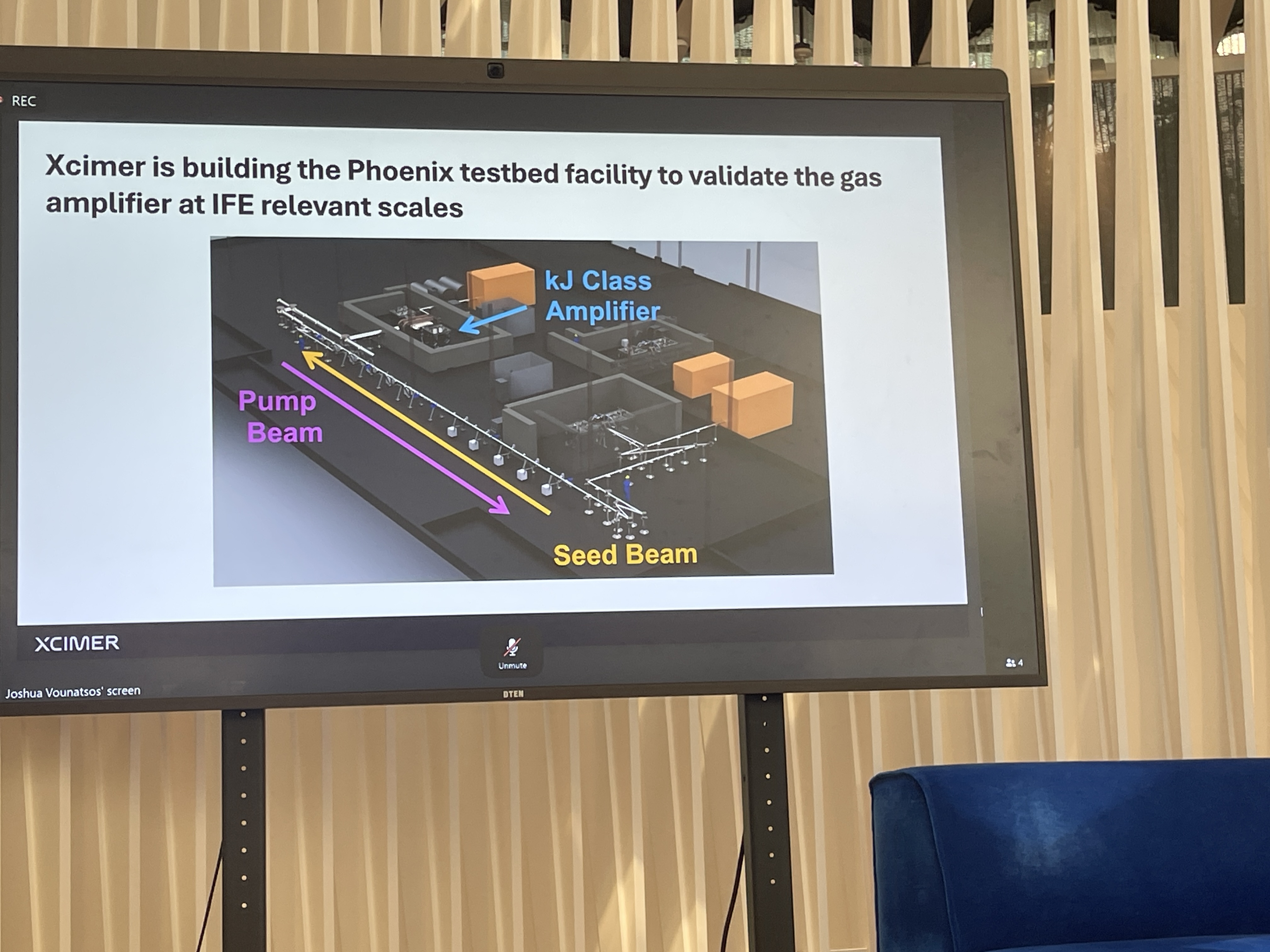
Why Invest in Fusion Energy?
Fusion energy presents a long-term opportunity for investors, but it remains a high-risk bet. The technology promises a nearly limitless energy source, using hydrogen isotopes—deuterium and tritium—found abundantly in water. Unlike nuclear fission, fusion produces helium as a byproduct, meaning no greenhouse gas emissions or long-lived radioactive waste. However, despite decades of research, commercial viability remains uncertain, and key challenges still need to be addressed.
Recent advancements have given the field a boost. Startups like Commonwealth Fusion Systems (CFS) and research at the National Ignition Facility (NIF) have demonstrated limited net energy gains—small but significant steps toward commercialization. While these breakthroughs have fueled optimism, fusion remains far from being a scalable energy solution. The technology still requires massive infrastructure, complex regulatory approvals, and fundamental scientific progress to become competitive with existing energy sources.
Scalability is another concern. Some startups focus on modular designs to decentralize energy generation for data centers, maritime power, and industrial facilities. While promising, these concepts are largely unproven outside of experimental settings. Unlike solar or wind, fusion requires expensive and highly specialized infrastructure, raising questions about how quickly it can be deployed at scale.
The Investment Opportunity
The field has seen a surge in private capital, with more than $5.5 billion in venture funding spread across 50+ startups worldwide. However, the vast majority of this funding has gone to a handful of well-capitalized companies like Commonwealth Fuel Systems and Helion Energy, which have been leading the way in proving how commercialization potential could play a role..
Global Policy and Funding Support
Government support for fusion is increasing, but still falls far short of what’s needed to bring the technology to market.
- United States – The U.S. House Committee recently passed an amendment regulating fusion devices as particle accelerators, a move that provides more regulatory flexibility but does not necessarily accelerate commercialization. In 2022, the U.S. announced a 10-year plan to consolidate fusion research under the Department of Energy (DOE), though funding remains limited.
- Germany – Announced €1 billion ($1.1 billion) for fusion research over the next five years, but this remains a fraction of what’s needed for full-scale deployment.
- United Kingdom – Allocated £650 million ($818 million) for fusion R&D and workforce training, though commercialization remains distant.
According to Dealroom.co, while funding peaked in 2021 due to major rounds for CFS and Helion Energy, 2023 saw more early-stage investments, signaling ongoing interest. However, fusion startups still face massive capital and regulatory hurdles, with most based in the U.S. (28 companies), Europe (13), Japan (3), Canada (2), Israel (1), and Australia/New Zealand (2).
The Reality Check for Fusion Energy
Fusion is often compared to Small Modular Reactors (SMRs)—a new class of compact nuclear fission reactors. While SMRs have existing regulatory pathways and proven technology, they still face cost and deployment barriers. Fusion, by contrast, operates in largely unregulated territory, giving startups more flexibility, but also more uncertainty.
For fusion to scale, companies will need to overcome land permitting, supply chain challenges, and a lack of specialized talent. Many key reactor components rely on suppliers from Japan, South Korea, and Italy, raising concerns about geopolitical and supply chain risks. The timeline for commercial fusion remains unclear—whether it arrives in 10 years or 50 years is still an open question.
Despite the excitement, fusion remains an unproven energy source. Investors should be cautious, recognizing that while the potential is enormous, the barriers to success are just as significant.
Below is the fusion energy landscape based on Dealroom data:

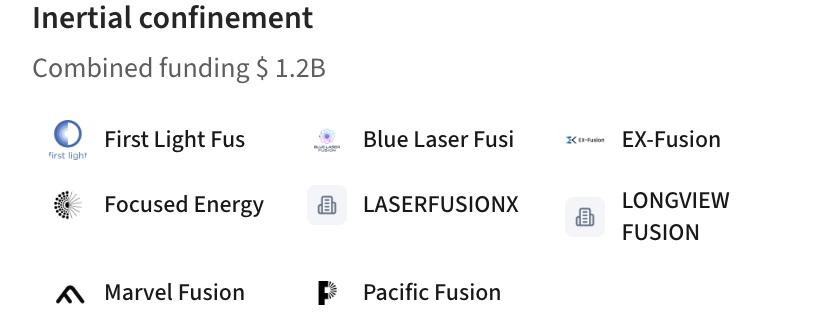

